34th Annual Meeting Program
Our members drive the NCM Annual Meeting program. NCM member perspectives, research, ideas and outlook ensure a rigorous meeting program while providing valuable contributions to ongoing research on motor control. Through a variety of topical multi-author sessions and individual presentations (oral or poster), the meeting provides a rare diversity of style and content that is unique and stimulating.
We look forward to welcoming all members of the NCM community to Panama City, Panama for the 34th annual meeting of the society.
Please note the times listed are in local time for Panama City (EDT).
List of Speakers
Join us in Panama to hear from the exciting confirmed speakers at NCM 2025!
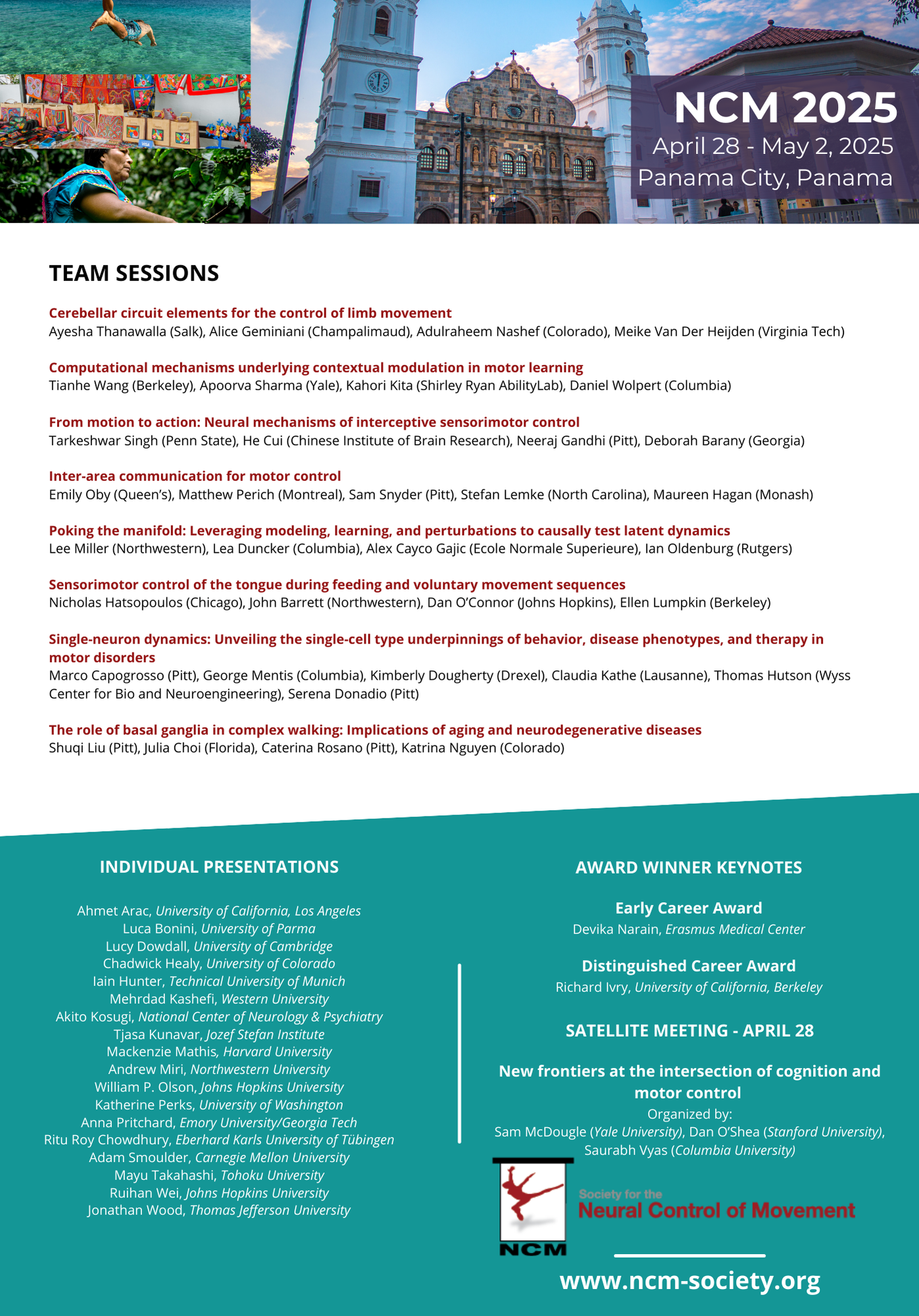
Detailed Program
Click on the tab for the day you’d like to learn more about. Further information will be added as it is confirmed.
08:00 – 17:30
Satellite Meeting
Join us for the satellite meeting “New frontiers at the intersection of cognition and motor control”. Find out more about the Satellite Meeting.
19:00 – 19:30
First timer social
Attending NCM for the first time? Join other first time attendees prior to the welcome reception. Key members of the NCM community, and members of the DEI committee, will be in attendance to welcome you to the meeting and walk with you over to the Opening Reception. A cash bar will be available for this informal networking event.
19:30 – 21:30
Opening reception
Join us to meet up with old colleagues and meet new ones at the opening reception. A full meal will be provided in an informal networking event with food stations and passed and plated appetizers. Join us at the Westin Playa Bonita Cantina (outdoor beach location) to kick off the annual conference!
08:00 – 10:00
Panel I - Cerebellar circuit elements for the control of limb movement
Ayesha Thanawalla 1, Alice Geminiani 2, Abdulraheem (Abed) Nashef 3, Amanda Brown4
1 Salk Institute for Biological Studies, 2 Champalimaud Center for the Unknown, 3 University of Colorado School of Medicine, 4 Baylor College of Medicine
Discussant: Eiman Azim
The ability to perform a wide repertoire of movements with precision and to maintain accuracy despite environmental changes relies on neural circuitry in the central nervous system. The cerebellum has long been known to play a critical role in controlling movement and adapting to environmental perturbations. The cerebellum is thought to refine and adapt movement through learning and implementing an internal model that predicts the outcome of motor commands, rapidly adjusts ongoing movement, and reduces error on subsequent actions. Crucial to its ability to learn, Purkinje cells in the cerebellar cortex receive instructive signals from climbing fibers that originate in the inferior olive and synapse onto Purkinje cells, whose dendrites are major sites for plasticity in the cerebellum. While the cerebellar cortex is thought to be the computational hub of the cerebellum, the cerebellar nuclei receive input from the Purkinje cells and, through its widespread connectivity, communicate with the brain and spinal cord, allowing the cerebellum to influence downstream motor structures. Defining how specific components orchestrate cerebellar learning and motor refinement at a circuit level benefits from a cell type- and pathway-specific approach. By describing recent advances in our understanding of the role of distinct cerebellar circuit elements, this panel aims to emphasize the insights into cerebellar function obtained through cell type-specific approaches in mice. Four speakers were selected for their ongoing work exploring distinct components of cerebellar circuitry. Alice Geminiani (Carey lab) will present optogenetic evidence that climbing fibers provide instructive signals for locomotor adaptation, thus being essential for cerebellar control of whole-body limb movement. Abed Nashef (Person lab) will describe a Purkinje neuron rate and synchrony code that is correlated with specific kinematic features during skilled reaching movements, shedding light on the interaction between Purkinje neurons and the cerebellar nuclei that facilitate online control of movement. Ayesha Thanawalla (Azim lab) will present work defining distinct cerebellar output networks and the rapid bidirectional control of forelimb kinematics and muscle activity by cerebellar nuclear neurons. Amanda Brown (Sillitoe Lab) will describe the neural activity of cerebellar nuclear neurons that distinctly represent cerebellar-associated motor disorders – tremors, ataxia, and dystonia. Eiman Azim will lead a panel discussion focussing on key themes, including: a) How cell-specific interrogation of neural circuits is revealing new insight into cerebellar function; b) How distinct cerebellar circuit elements may function in motor learning vs motor execution; c) The degree to which disruption in any of these pathways influences cerebellar-linked movement disorders; d) how to facilitate increased interaction between those studying cerebellar function in mice and primates.
10:00 – 10:30
Coffee Break
10:30 – 11:05
Early Career Award Talk - Devika Narain
Timing and motor control are inexorably linked, and from this interplay emerge various feats of motor precision that pervade the animal kingdom. Yet, laboratory measurements of the timing of movements are riddled with variability and biases that paint a contrasting picture. Previous work has sought to resolve this conundrum using Bayesian theory, which formalizes how prior beliefs about temporal variables shape precise actions under environmental uncertainty. While these frameworks account for various behaviors in different domains, the neural mechanisms underlying the acquisition and use of such prior beliefs continue to elude us. Here, we propose a role for cerebellar circuits in acquiring prior knowledge to shape rudimentary predictive motor behaviors, such as the conditioned eyelid response in Pavlovian eyeblink conditioning. We will show evidence that cerebellar Purkinje cells encode probability distributions of the stimuli and propose a mechanism for how this manifests in the kinematics of the motor output. Furthermore, at the population level, we will show that cerebellar cortical dynamics assume a topological organization in the form of curved manifolds, which encode prior knowledge along the curvature of these topologies. In the second part of the talk, we will present methodological work that aims to unravel topologies and embed these in their intrinsic dimensions to decode task-relevant information. Towards the end, we will use this method to test the hypothesis that the curvature of neural manifolds encodes prior knowledge in sensorimotor timing tasks. Overall, we will propose a neural mechanism for how prior beliefs for the temporal control of movements are acquired by neurons and eventually encoded in the topologies of populations, while adhering to the predictions of normative theory that explain the emergence of precise sensorimotor timing behaviors.
11:05 – 13:05
Individual I
OS1.1 – Neuroethology of bodily and manual actions in freely moving monkeys
Luca Bonini 1
1 University of Parma
Presenting Author: Luca Bonini
Decades of brain research on the cortical motor system have leveraged nonhuman primates to investigate the anatomo-functional organization of voluntary, goal-directed actions. However, the concept of “goal” and its relationship to the voluntary nature of an action remain widely debated. Studies using intracortical microstimulation (ICMS) have revealed that the frontal motor system encodes final postures of multiple body parts resembling those seen in natural actions. Single-neuron recordings suggest that premotor neurons encode the achievement of specific outcomes, such as reaching a spatial location or obtaining a piece of food, often independently of the sequence of extension-flexion movements or even the effector used to achieve them. This supports the idea that goal coding is a key organizational principle of the premotor cortex. However, these studies are derived from laboratory experiments in restrained contexts (RCs), under the assumption that this knowledge generalizes to freely moving contexts (FMCs). To date, no study has directly investigated the premotor control of natural actions in freely moving monkeys.
To fill this gap, we developed a neuro-behavioral platform enabling stepwise wireless recording of the same premotor neurons in both RC and FMC while filming two monkeys’ behavior with a multicamera system. We found that neurons often encoded the same hand and mouth actions differently in RC and FMC. Furthermore, in FMC, we identified cells selectively encoding actions untestable during RC and others displaying mixed selectivity for multiple actions, consistent with an organization based on cortical motor synergies rather than action goals at different levels of complexity. Interestingly, cross-context decoding of the actions testable in both contexts demonstrated that neural activity in FMC is richer and more generalizable to RC than vice versa, possibly due to the coordinated control of head movement with specific effectors, such as the hand and the mouth, as revealed by head-free ICMS. During reaching-grasping actions directed at objects of different sizes in FMC, we could accurately decode both head movements and grip types, suggesting the existence of previously overlooked synergistic control of distal and proximal motor components in the premotor cortex underlying the flexible organization of primate natural actions.
Our findings support the relevance of neuroethological approaches in unveiling the neural bases of spontaneous, natural behaviors.
OS1.2 – Motor cortical influence during ethological motor behavior
Andrew Miri 1, David Xing 1, Amy Kristl 1, Natalie Koh 1, Zhengyu Ma 1
1 Northwestern University
Presenting Author: Andrew Miri
It remains poorly resolved when and how motor cortical output directly influences muscle activity through descending projections. The involvement of motor cortex in motor learning and movement preparation complicates the interpretation of lesion and other inactivation results vis-à-vis movement execution, as disturbance to processes on which execution depends can impede execution itself. Direct motor cortical influence could take several possible forms: motor cortex could drive the totality of limb muscle activity patterns, it could participate in unison with the rest of the motor system in generating motor output without playing a necessary role, or it could selectively influence particular components of muscle activity, such that it informs (“instructs”) ongoing muscle activity pattern.
To address when and how motor cortical output directly influences muscle activity, we combined optogenetic silencing, electromyography, and Neuropixels recording in mice performing a naturalistic climbing behavior where they effectively traverse an unpredictable terrain. We found that during climbing, the caudal forelimb area (CFA, rodent homolog of forelimb M1) informs muscle activity pattern, acting mainly by selectively exciting certain muscles while exerting smaller, bidirectional effects on their antagonists. Analysis of Neuropixels recordings identified components of CFA activity that correlate with its short latency influence on muscles. These components partially overlap with those that correlate with muscle activity, but are entirely different from those that correlate with forelimb kinematics. This suggests that selective motor cortical influence does not rely on the activity components the field has traditionally focused upon. We also discovered that a substantial fraction of CFA neurons are primarily active during a very limited subset of the forelimb muscle activity states that occur during climbing. This is analogous to the firing of many hippocampal neurons only at particular locations within an environment. These CFA firing patterns are not consistent with the idea that motor cortical activity is well-described as a low-dimensional linear dynamical system.
We have also extended our approach to mice freely exploring an arena that elicits a broad range of naturalistic behaviors, including stereotyped, species-typical behaviors like eating and grooming, and those that challenge agility and dexterity like climbing and walking on an irregular grid. Results here suggest that selective direct motor cortical influence extends to a broad range of naturalistic behaviors. We have also found that CFA’s influence on muscles during climbing is substantially larger than during single-forelimb reaching, suggesting a substantial behavioral dependence of direct motor cortical influence. Other results indicate that this variation across behaviors extends to CFA’s interactions with the rostral forelimb area, the rodent homolog of forelimb premotor cortex, as well.
OS1.3 – Motor cortical dynamics during reaching connect posture-specific attractors
Mehrdad Kashefi 1, Jonathan Michaels 2, Joern Diedrichsen 1, J. Andrew Pruszynski 1
1 Western University, 2 York University
Presenting Author: Mehrdad Kashefi
One of the central questions in motor neuroscience is how the population of motor cortical neurons control voluntary movement. Much of our current understanding of this question is based on so-called center-out reaching tasks that involve reaching from a single start location to various spatial targets — a situation that confounds reach direction with limb posture at the spatial target. Because of this confound, previous studies lack the required condition variability to probe the geometry of neural dynamics (NDs) for movements across different postures and extents. To address this confound, we trained two macaques to move their arm between all possible combinations of five targets located on the vertices and at the center of a rectangle in a 2D exoskeleton (KINARM). This target geometry allowed us to dissociate the abstract cue of the final target from the direction of the reach in certain trial types. For example, diagonal reaches included movements with the same start location and in the same direction but with different extents. Similarly, some reaches shared the same final target and direction but began from different starting locations. We used Neuropixels probes to record single-unit activity from multiple brain regions in two monkeys. In Monkey M, we recorded from primary motor cortex (M1; N=962) and dorsal premotor cortex (PMd; N=833). In Monkey P, we recorded from M1 (N=1310), PMd (N=620), the supplementary motor area (SMA; N=590), pre-SMA (N=310), dorsolateral prefrontal cortex (dlPFC) (N=380), and the internal segment of the globus pallidus (GPi, N=549). In both monkeys, in M1 and PMd, we observed an elegant compositional arrangement of NDs for movement with different postures, extents, and directions with two striking features. First, a posture subspace with attractor points for each spatial target. These attractors were visited whenever the arm rested in its respective target before or after the reach. Second, rotational dynamics that linked the attractor points. These rotational patterns were aligned such that more similar rotational dynamics were associated with more similar reach directions. In monkey P, we could decode start and end locations and movement extent from all recorded sites, though dynamics’ geometry differed significantly across regions. To gain mechanistic insight, we trained recurrent neural networks (RNNs) to control a biomechanical arm in a 2D workspace and analyzed their NDs in the same 5-target task. A posture subspace emerged consistently across training parameters. However, RNN dynamics most closely resembled M1/PMd when two factors were applied: (1) regularization of hidden activity smoothness and (2) a spinal RNN module between the main RNN and the arm model. Our work provides fundamental insight into the geometry of NDs in the primate motor cortex during self-initiated reaching, with important implications for brain-computer interface design.
OS1.4 – Conditioning of limb impedance affects adaptation to novel dynamics
Iain Hunter 1, Sae Franklin 1, Raz Leib 1, David Franklin 1
1 Technical University of Munich
Presenting Author: Iain Hunter
Adaptation to changes in our environment is accompanied by increased muscle co-contraction. This increases the mechanical impedance of the limbs, which resists changes in the environment until a more efficient control strategy, such as a predictive controller, is learned. Increased co-contraction is particularly important for stabilizing motion in unstable and unpredictable environments. However, the impact of co-contraction on adaptation and generalization is relatively understudied. Heald et al., (2018) used explicit commands to increase co-contraction and showed that this accelerates motor learning, but did not examine the underlying mechanism. Here we examine how prior conditioning of co-contraction affects the adaptation to novel dynamics, and the generalization of the motor memory.
Participants were randomly assigned to either high or low impedance groups. Electromyographic (EMG) activity was recorded from six muscles (three antagonistic pairs) throughout the experiment. Participants grasped the handle of a robotic manipulandum and performed 2,450 reaching movements. The protocol included pre-exposure (450 trials in the null field (NF)), conditioning, and exposure phases. During the conditioning phase, participants performed 1000 trials to a single target either in the NF (low impedance group), or in a divergent force field (high impedance group) to promote changes in muscle co-contraction. Finally, all participants performed 1000 reaches to a single target (0°) in a counterclockwise curl field (CF). Force channel trials were interspersed throughout the pre-exposure, conditioning and exposure phases, to enable measurement of force compensation and generalization.
Prior conditioning successfully altered the co-contraction in each group, causing differences in early adaptation to the force field. The high impedance group produced small kinematic errors, relative to those generated by the low impedance group. The low impedance group demonstrated higher variability of adaptation, however, both groups had similar speeds of adaptation to the force field. This may be due to a rapid increase in co-contraction in the low impedance group. Interestingly, the difference in the initial errors affected the spatial generalization of learning. Participants in the high impedance group exhibited more localized adaptation compared to the low impedance group, who generalized across a wider range of target directions. This suggests that increased impedance enhances error correction in the state space experienced during training, consistent with the motion-referenced learning framework. Overall, our work demonstrates that increased impedance narrows the generalization of motor memory.
OS1.5 – Population dynamics in the motor control and restoration of head stabilization driven by vestibular pathways during locomotion
Ruihan Wei 1, Oliver Stanley 1, Adam Charles 1, Kathleen Cullen 1
1 Johns Hopkins School of Medicine
Presenting Author: Ruihan Wei
Vestibulospinal reflexes are essential for maintaining balance and head stability during locomotion. Individuals with vestibular sensory loss suffer from severe gaze instability and postural imbalance, significantly impairing their mobility. The vestibulo-collic reflex in particular, which activates neck muscles in response to head movement, is thought to play a central role in stabilizing the head during locomotion. We thus sought to better understand vestibular contributions to head stability and motor control strategies across different locomotion contexts, as well as to determine the effectiveness of prosthetic vestibular stimulation at restoring function. We recorded single and multi-motor unit activity in the splenius capitis and sternocleidomastoid muscles using intramuscular EMG in normal monkeys, monkeys with complete bilateral vestibular loss (BVL), and BVL monkeys during head-coupled vestibular prosthetic stimulation. Head and trunk positions were tracked using a head-mounted 6D motion sensor and marker-based tracking systems, while limb movements were analyzed using high-speed video and DeepLabCut. Single and multi-motor unit activity and head stabilization were evaluated during overground and treadmill walking at various speeds. Principal components analysis was applied to the EMG data to quantify population muscle activation for each locomotor context.
In normal monkeys, neck motor unit recruitment was gait phase-locked and antagonistic, facilitating effective head stabilization under various walking conditions. Principal component analysis revealed a consistent activation geometry that scaled with speed during treadmill walking. In contrast, speed-matched overground walking displayed a distinct geometry with heightened neck muscle responses and enhanced head stabilization. Following bilateral vestibular loss, monkeys exhibited pronounced head oscillations and inadequate compensation for body motion, with reduced phase-locking of neck muscle activity. BVL monkeys failed to adopt a consistent strategy across speeds, although overground walking retained a unique activation geometry compared to speed-matched treadmill locomotion. With head-coupled vestibular prosthetic stimulation, the head motion became more stable, and the gait phase-dependent modulation of neck muscle recruitment was restored. Population-level muscle activity regained a motor control strategy again displaying a consistent activation geometry that scaled with speed during treadmill walking and a unique activation geometry during overground walking.
In summary, our results first reveal the presence of population-based motor strategies within and between conditions during simple, innate behaviors, which enable flexible control of head stabilization. In addition, our findings demonstrate the critical role of the vestibular system in coordinating neck motor activity to generate compensatory head movements, ensuring head stabilization in space during locomotion. Finally, our results indicate that prosthetic stimulation can restore the control strategies that the brain uses to maintain balance and head stability during natural behavior, which may provide a foundation for advancing the treatment of vestibular dysfunction by improving strategies for restoring effective locomotion in affected individuals.
OS1.6 – Hierarchical model balancing efficiency and safety in human motor control
Tjasa Kunavar 1, Jan Babic 1, Erhan Oztop 2, Mitsuo Kawato 3
1 Jozef Stefan Institute, 2 Ozyegin University, 3 ATR Brain Information Communication Research Laboratory
Presenting Author: Tjasa Kunavar
Our research presents a novel perspective on motor learning, exploring how ecological fitness (organism’s specific traits and abilities that aid survival in its environment) factors into human motor control. Our sensorimotor system is crucial for ecological fitness since it enables adaptive movement control vital for survival. We delve into how ecological factors contribute to survival by enhancing movement efficiency, while considering the risk of injury associated with failure. Existing motor control theories, which primarily focus on isolated body movements, often neglect these ecological aspects. By redefining computational motor control optimality to incorporate ecological fitness, we propose a strategy that alternates between success-driven efficiency and failure-driven safety. This insight was made possible by an experimental paradigm specifically designed to challenge the sensorimotor system with the realistic possibility of failure and injury risk, underscoring the robustness of the adaptive strategies we identified. Our experimental paradigm involved whole-body squat-to-stand motions with novel backward force perturbations. An integral part of the learning process were failures – movements when the participant lost postural balance and had to make corrective steps to prevent a fall. Participants adapted to the perturbed squat-to-stand movements and dramatically reduced failures, while showing various adaptations to prevent falls. We show that computational motor learning mechanisms of the brain are not flat and utilize hierarchical organization for efficiency, adaptability and robustness. Our data suggests a top-level ecological controller in human motor learning, optimizing for safety or efficiency based on failure or success, to form motor plans to be used by lower-level control. Adaptation of motor plan occurs through fast reinforcement learning mechanism after failed execution of motion. To achieve the intended plan, motor control processes encompass internal model learning and feedback gain tuning mechanisms in addition to the feedback control. Adaptation of motor control is slower and predominantly occurs as a result of adaptation of internal model during movement execution irrespective of movement success. This new model provides a more holistic view of human motor control, integrating risk management in a hierarchical learning system applicable to ecological situations.
13:05 – 15:30
Posters, Exhibitors and Lunch
15:30 – 17:30
Panel II - From motion to action: Neural mechanisms of interceptive sensorimotor control
Tarkeshwar Singh 1, He Cui 2, Neeraj Gandhi 3, Deborah Barany 4
1 Pennsylvania State University, 2 Chinese Institute for Brain Research, Beijing, 3 University of Pittsburgh, 4 University of Georgia
Discussant: John Douglas Crawford
Interceptive movements, from catching a ball to chasing a toddler running towards the street, represent a complex class of motor behaviors that provide an ideal paradigm for investigating fundamental questions in motor control. Unlike stationary targets, moving targets fundamentally transform sensorimotor demands by requiring precise spatiotemporal coordination, continuous integration, and adaptive error correction. This session brings together four animal and human research programs examining how the brain processes motion signals to guide interceptive movements. The speakers present approaches spanning non-human primate neurophysiology, human TMS, EEG, and behavioral studies to unravel the neural computations underlying interception.
He Cui will present pioneering work in non-human primates performing manual interception of circular moving targets. Their findings reveal that monkeys accurately compensate for both sensory and motor delays during interception. Single-unit recordings demonstrate that posterior parietal cortex encodes planned movement parameters rather than instantaneous visual information, while motor cortex exhibits mixed selectivity for both movement planning and ongoing target motion. Novel analyses of population-level neural dynamics suggest orthogonal encoding of target velocity in motor cortical activity, supporting robust forward predictions for motor planning and timing control.
Raj Gandhi will discuss how the brain overcomes neural processing delays using the saccadic system as a model. Through integrated human behavioral and monkey neurophysiology studies, his work reveals systematic variations in interceptive saccade parameters based on target motion features. Superior colliculus recordings demonstrate that target motion reshapes classical receptive field properties and alters neural response profiles, providing insight into early sensorimotor transformations for interception.
Deborah Barany will present TMS investigations of how visual motion information shapes motor cortical output during interceptive movement preparation. Her studies reveal that corticospinal excitability is selectively enhanced prior to intercepting faster-moving targets, independent of target distance or eye movement strategy. This modulation appears specific to movement preparation rather than motion perception, providing direct evidence for how target motion parameters influence motor system excitability.
Tarkesh Singh will present research on mechanisms of motion processing for postural control and interception. His studies reveal that while young adults utilize both retinal and extraretinal motion signals for posture stabilization, older adults rely predominantly on extraretinal mechanisms. EEG findings demonstrate connectivity between motion-processing areas and premotor regions during motor planning. His work also highlights how extraretinal signals modulate long-latency reflexes to stabilize posture during interactions with moving objects.
17:30 – 18:30
Trainee social
Sponsored by
All trainees welcome to join us for a casual, networking social following the conclusion of the day. Network in a casual environment, get to know new people, and enjoy this trainee focused event.
08:00 – 10:00
Panel III - Poking the manifold: Leveraging modeling, learning, and perturbations to causally test latent dynamics
Lee Miller 1, Lea Duncker 2, Alex Cayco Gajic 3, Ian Oldenburg 4
1 Northwestern University, 2 Columbia University, 3 École Normale Supérieure, Paris, 4 Rutgers University
Discussant: Matthew Perich
100 years after Bernstein’s pioneering efforts to understand the brain’s control of redundant motion, interest has focused on analyses of “neural manifolds”, low-dimensional representations of neural activity which result from the redundancy within the brain itself. This approach has led to new insights about motor preparatory activity and the relationship between neural and behavioral dynamics. In addition to the ability to record exponentially larger numbers of neurons, the past decade has ushered in tremendous advances in optogenetics, as well as AI-inspired neural network models that link task computation to latent dynamics and behavior. These complementary approaches to understanding the relation between neural activity and behavior go well beyond the limitations of correlational analysis of recorded activity.
Miller will begin by providing an overview of the concepts and analytical approaches needed to understand the discipline. He will present data demonstrating the remarkable stability between low-dimensional, latent signals and well-learned behavior. These include data recorded during learning, and in the cage, where the physical constraints of the lab setting are eliminated. The dynamics of the latent signals differ to the extent that linear decoding of EMG fails across the contexts, but the results shed light on how these behaviors are controlled. Next, Cayco Gajic will present modeling studies examining the tension between the need for a stable representation of behavior, while still enabling rapid adaptation with learning. She will describe a multi-region RNN model of the interaction between the motor cortex and cerebellum, capable of learning a visual motor adaptation task, and propose an optogenetic experiment to test its validity. Then, Duncker will describe work combining computational modeling with analyses of neural population responses to optogenetic and ICMS perturbations during reaching. This approach allows her to evaluate hypotheses about the network-level dynamical mechanisms underlying pattern generation in motor cortex. She will show that stimulation in M1 perturbs reaching only to the extent that it alters neural states within an identifiable low-dimensional dynamical subspace. Finally, Oldenburg will describe his pioneering work using holographic optogenetics to control the activity of hundreds of neurons in behaving mice with millisecond precision, allowing him to evoke user-designed population vector activity, and to test predictions in actual neuron networks. Compared to ICMS or typical optogenetic methods, holographic control of network activity promises the ability to manipulate network activity with unprecedented precision.
We seek to engage the audience in a discussion of the strengths and weaknesses of the recording, stimulation, and modeling approaches, and the necessary next steps to advance the field of neuronal manifolds to understand the causal connections linking circuits to activity to behavior.
10:00 – 10:30
Coffee Break
10:30 – 12:30
Individual II
OS2.1 – Motor adaptation in redundant input spaces occurs through changes in both internally-generated feedforward control and sensory-driven feedback control
Katherine Perks 1, Lydia Smith 1, Sam Burden 1, Amy Orsborn 1
1 University of Washington
Presenting Author: Katherine Perks
Introduction: To control a familiar motor effector, like a computer mouse, we rely on both sensory information (feedback control) and internal predictions about how the effector works (feedforward control). But how do we adapt to unfamiliar effectors? Human psychophysics studies have identified multiple types of motor adaptation using visuomotor perturbations. However, these experiments often focus on assessing changes in feedforward control and lack methods to simultaneously quantify feedback control. They also use simplified tasks where each dimension of movement is associated with a unique dimension of feedback, which does not reflect the redundancy inherent in natural motor tasks and effectors. Redundancy may create challenges for learning as not all input changes produce distinct feedback. We hypothesize that adapting both feedforward and feedback control is critical to learning redundant sensorimotor transforms. We designed a novel paradigm that combines visuomotor perturbations in a rich input space with a control theory-based task that disentangles the two forms of control (Yamagami 2021; Yang 2021). This allowed us to study feedforward and feedback control changes during input-redundant motor learning in non-human primates for the first time.
Methods: We trained two male rhesus macaques to control the movement of a 1D cursor using unconstrained 3D hand movements. We specified an ‘intuitive’ initial control axis in 3D space Mi onto which hand position was projected and transformed into cursor position. The monkeys used Mi to perform a 1D tracking task, which consisted of following a pseudo-random moving target while also correcting for a disturbance applied to the cursor. The target and disturbance trajectories contain distinct frequencies, which enables separate quantification of feedforward and feedback control. Once the monkeys were well-trained on the task using Mi, we leveraged the redundant input space to create perturbed control axes Mp, which rotated Mi by 20°, 60°, 90° or 180° (a total of N=8 perturbations, complete in one monkey and in progress in the other). As the monkeys adapted to the perturbations, we recorded their movements and quantified their feedforward and feedback control to link changes in each controller to changes in behavior and performance.
Results: Monkeys adapted to all Mp but adapted faster as they were exposed to more perturbations. This suggests they learned not only the particular Mp but also general learning strategies. Surprisingly, the 180° rotation required the most time to learn, despite sharing the same task-relevant axis as Mi. We found the monkeys used many different strategies to increase the amount of feedback received, including making larger movements early in learning to compensate for less overlap between their movements and Mp. We found performance gains correlated with improvements in feedforward control as well as aspects of feedback control, which has not previously been reported. This supports our hypothesis that motor learning in redundant input spaces involves updating both internally-generated predictions and the way feedback signals are used. Our results provide novel insight into how motor adaptation occurs in previously unstudied contexts and will enable new ways to study the neural mechanisms of motor learning.
OS2.2 – Region-specific neural dynamics and interactions during complex dexterous movements
Ahmet Arac 1, Sanjay Shukla 1, Erica Nagase 1, Alan Yao 1, Nicolas Jeong Lee 1, Kate Santoso 1, Emily Stenzler 1, Kasey Kim 1, David Lipkin 1, Angela Kan 1, Christina Abdishoo 1
1 University of California, Los Angeles
Presenting Author: Ahmet Arac
Complex motor actions, such as reach-and-grasp movements, rely on the coordinated activity of multiple brain regions, each contributing distinct computations to control behavior. To uncover the neural principles underlying this process, we recorded spiking activity across 10 brain regions (primary and secondary motor and primary sensory cortices, multiple regions in caudateputamen, globus pallidus externa and interna, motor nucleus of thalamus, and deep cerebellar nucleus) in mice performing a skilled reach-and-grasp task. Through detailed kinematic analysis, we identified four distinct behavioral phases: a preparatory phase, two movement phases, and a post-grasp phase. Each phase exhibited unique kinematic parameters, highlighting the modular nature of the movement.
Single-neuron analyses revealed that while similar proportions of neurons (60–80%) across regions responded during each phase, the strength of these responses varied. Notably, response strengths were consistently lowest during the first movement phase across all regions, with region-specific variations emerging in subsequent phases, particularly in the primary sensory cortex (S1). Despite these similarities in single-unit activity, dynamical systems modeling revealed distinct, phase-specific computational rules across regions. Using recurrent switching linear dynamical systems (rSLDS) modeling, we demonstrated that neural state transitions aligned closely with behavioral phase transitions in the primary motor cortex (M1) and secondary motor cortex (M2). However, this alignment was less consistent in other regions, underscoring region-specific computational dynamics. The properties of the dynamical rules were also different across phases and regions.
To explore inter-regional interactions, we implemented data-driven recurrent neural network (RNN) modeling combined with in silico perturbation experiments. These analyses highlighted a specialized role for M2 in modulating M1 activity during the pre-grasp phase. This finding was confirmed through optogenetic inhibition of M2 during neural recordings in M1, which disrupted the reach-and-grasp behavior when applied before the grasp but had no effect afterward. Further, RNN-based pairwise inhibition experiments revealed phase-specific influences of other regions on M1, demonstrating a dynamic and context-dependent hierarchy within the motor network.
Additional analyses of trajectory tangling provided insight into neural variability across phases. S1 exhibited significantly higher tangling (as shown before) across trials compared to other regions, while other regions exhibiting low tangling. Intriguingly, M1 tangling remained consistently low across phases, while M2 tangling progressively increased, suggesting a differential role for M2 in shaping motor output.
In summary, our results demonstrate that reach-and-grasp behavior is composed of kinematically distinct phases, each governed by unique neural computations and inter-regional interactions. While single-unit responses often appeared similar across regions, the underlying dynamical rules were highly region- and phase-specific. These findings reveal a sophisticated interplay of hierarchical and parallel processing within the motor network, advancing our understanding of how different brain regions coordinate to execute complex motor tasks.
OS2.3 – Hierarchical state decoding for seamless selection between multiple iBCI functions
Anna Pritchard 1, Samuel Nason-Tomaszewski 1, Brandon Jacques 1, Yahia Ali 2, Kaitlyn Tung 3, Payton Bechefsky 4, Leigh Hochberg 5, Nicholas Au Yong 1, Chethan Pandarinath 1
1 Emory University and Georgia Institute of Technology, 2 Georgia Institute of Technology & Emory University, 3 Georgia Institute of Technology, 4 Coulter Department of Biomedical Engineering, Emory University and Georgia Tech, 5 Brown University
Presenting Author: Anna Pritchard
Intracortical brain-computer interfaces (iBCIs) have demonstrated significant potential for restoring control of movement and speech for people with tetraplegia and dysarthria. In previous demonstrations, iBCI users had arrays placed targeting a single control modality (brain areas canonically associated with hand or speech). Recently, BrainGate2 Clinical Trial participant T16 had 4 microelectrode arrays placed in precentral gyrus (PCG) targeting hand (Brodmann’s area 6d) and speech (6v&55b) modalities. Recording from multiple brain networks enables multifunctional control, but usability is severely limited without the ability to switch between functions (controllable outputs within a modality), as alternatives require either constant activation of all functions or manual switching. Here, we investigate the potential for a hierarchical state decoder to infer the iBCI user’s intended functional output. Inspired by at-home iBCI computer use where cursor control may not be a constant intent, we first designed a cursor-and-click task during which T16 was randomly prompted to use various hand or speech functions (e.g. move pinky, speak, think, do nothing). We identified channels that encoded specific functions (e.g. channels modulated differently for think vs say ‘bring’, or move pinky up vs control cursor with pinky). Trials were classified by condition from all (71% acc. all 9 conds.), hand (75% 4 hand conds.), and speech (67% 5 speech conds.) arrays. This suggests that PCG shows distinctions between not only hand and speech modalities, but also distinct functions within hand and speech. Next, we designed a multifunctional iBCI task where T16 was instructed to sequentially read a sentence, use brain-to-text to type the sentence, and use the cursor iBCI to select incorrect text predictions. Offline, we modeled the data with Hidden Markov Models (HMMs) to distinguish task phases (idle, read, speak, cursor). We compared individual hand- and speech- array HMMs against a single HMM using all arrays for classifying all functions (all/speech/cursor acc. 62%/90%/78% single; 54%/89%/72% dual). Finally, we demonstrated the feasibility of these HMMs to decode T16’s functional intent in an online iBCI setting. T16 performed a similar multifunctional task as before, but the cursor and speech decoders were de/activated according to the decoded user intention. Speech (90% acc.) and cursor (70%) classification reflect offline results with minimal latencies for decoded speech onset/offset (-0.83+/-0.73s onset, 0.32+/-0.43s offset). Next we will demonstrate the use of state decoders for switching between functions within a modality (cursor and robot) and integrate function-switching with personal iBCI use. These decoders enable iBCI users to independently switch control between multiple modalities, may enable control of multiple functions within a modality, and can be extended to facilitate multifunctional iBCI control across a multitude of applications.
OS2.4 – A novel peripheral neural interface to manipulate limb movement through myocontrolled optogenetic sensory nerve stimulation
Akito Kosugi 1, Moeko Kudo 1, Shiro Egawa 1, Ken-Ichi Inoue 2, Masahiko Takada 2, Kazuhiko Seki 1
1 National Center of Neurology and Psychiatry, 2 Kyoto University
Presenting Author: Akito Kosugi
Neural interfaces targeting the peripheral nervous system, such as cochlear implants, are primarily designed to restore lost sensory perception, with relatively few addressing motor functions. While functional electrical stimulation has been used to restore motor functions, it often faces challenges in adapting to dynamic movements due to the difficulty in modulating multiple muscle activities. In this study, we focused on proprioceptive reafferent signals that are generated by one’s own movement (reafference). These signals not only contribute to somatosensory perception through transmission to the somatosensory cortex, but also play a crucial role in generating muscle activity via the spinal reflex loop. Since these signals are distributed throughout the spinal cord, their modulation can influence multiple muscle activities through the reflex loop, offering the potential to correct inappropriate dynamic movements. In addition, this event could occur without the need for volitional motor commands, thereby reducing a cognitive effort to restore motor functions. Here, we propose a novel peripheral neural interface that targets the somatosensory reafferent signal to restore motor functions. Using optogenetics, we specifically modulated peripheral sensory nerve activity during animal’s reaching movements to examine the effects of neuromodulation on ongoing movements.
First, we investigated the optimal serotype and administration route of adeno-associated virus (AAV) vectors for selective targeting of large-diameter afferents associated with tactile and proprioceptive sensations in common marmosets. We found that systemic administration through intravenous injection of the capsid variant of a serotype-9 AAV vector (AAV-PHP.B) selectively transduced channelrhodopsin 2 (ChR2) into large-sized dorsal root ganglion (DRG) neurons. In acute electrophysiological experiments, we confirmed the selective activation of large-diameter afferents via optical stimulation to the peripheral nerve.
Next, we trained animals to perform a visually-guided reaching task. After completing the training, we transduced ChR2 into large-sized DRG neurons. During task performance, we applied transdermal optical stimulation to the afferent nerve from the wrist flexor muscles (median and ulnar nerves). When we applied the stimulation by triggering the EMG activity of the agonistic muscle of reaching (triceps brachii muscle), animals exhibited undershot reaching and failed to reach the target. Further analysis revealed that this undershooting was caused by reduced activity in the triceps brachii muscle, which was due to the recruitment of reciprocal inhibitory reflex induced by optogenetic stimulation. These results highlight the significant impact of optogenetic sensory nerve stimulation on ongoing movement. Furthermore, this suggests the potential of a novel peripheral neural interface that targets peripheral sensory nerves to correct overshooting limb movement, such as dysmetria.
OS2.5 – Developing a sensory representation of an extra robotic digit
Lucy Dowdall 1, Giulia Dominijanni 2, María Molina 1, Edmund Da Silva 1, Fumiya Lida 1, Matteo Bianchi 3, Dani Clode 1, Tamar Makin 1
1 University of Cambridge, 2 École Polytechnique Fédérale de Lausanne, 3 University of Pisa
Presenting Author: Lucy Dowdall
Our motor system relies on somatosensory feedback to inform about the state of our limbs and interactions with our environment. This creates challenges for wearable technology that interface with our sensorimotor system, as they are thought to have ‘open-loop’ control, with no direct tactile feedback available. However, a neglected area is the natural sensory feedback we can receive from how such devices are worn on, and controlled by, our bodies.
When using an extra robotic finger – the Third Thumb (Dani Clode Design), worn on the side of the hand (the ‘palm’), participants can successfully develop a sense of proprioception (Kieliba et al., 2021). This implies the natural sensory feedback can be used to inform about device ‘somatosensation’.
To characterise how this natural feedback is experienced, we identified two key components – skin stretch of the palm when the Thumb moves and vibrotactile information when objects engage with the Thumb tip. We then developed two artificial feedback systems for use with the Thumb that replicated these aspects (stretch and vibration) to offer comparison between natural feedback and artificial touch technology. Participants (N=20) performed a softness discrimination task (where we expected the skin stretch to excel) and a texture discrimination task (where we expected the vibrotactile to excel) with the natural and artificial systems. People could successfully perform the tasks with the artificial systems, however they performed comparably with the natural feedback, demonstrating we can extract meaningful information from it.
To then explore how our somatosensory system processes this information, we used fMRI to study the representational similarity patterns across the hand and Thumb in primary somatosensory cortex (S1) (N=50). We found that after only limited exposure, the brain already organises this tactile input from the Third Thumb in a topographically appropriate manner and in a distinct way from the palm.
Next, we examined how this natural tactile input supports motor learning. Participants completed 7 days of Thumb-hand collaboration training (n=30), whilst controls received 7 days of keyboard training (n=20). Following this altered finger-synchronisation motor training, we observed reduced inter-finger information content in S1, with larger changes in the Third Thumb group. Importantly, Thumb training produced specific increases in representational similarity between the Third Thumb and biological hand. This integration implies construction of a sensory representation of the Third Thumb in relation to the fingers through their co-usage.
Overall, we have demonstrated the utility of tactile feedback received as a natural by-product of how technologies interface with our body. Our brain can access a sensory representation after only limited exposure to such feedback, which can be integrated with our body following training. Such sensory cues could provide a way of ‘closing’ the sensorimotor loop.
OS2.6 – Neuro-musculoskeletal modeling reveals muscle-level neural dynamics of adaptive learning in sensorimotor cortex
Mackenzie Weygandt Mathis 1
1 EPFL
Presenting Author: Mackenzie Mathis
The neural activity of the brain is intimately coupled to the dynamics of the body. In order to predict the sensorimotor consequences of our actions, compelling behavioral studies in humans, non-human primates, and in rodents have shown the existence of internal models — predictive models of our body in the environment. However, the neural computations that update these internal models during motor learning remains largely unknown. There is growing evidence in several sensory areas, such as in the visual and auditory primary cortex, that activity of layer 2/3 encodes sensory prediction errors when unexpected stimuli are presented, which theoretically could serve as a teaching signal. Notably, somatosensory (S1) is essential in rodents and humans learning to adapt arm movements, but, if equivalent prediction errors are observed in S1 has not been established. Lastly, an outstanding question more generally for prediction errors is in which coordinate frameworks would such errors be computed? Here, we directly test whether errors are encoded in S1 during a motor adaptation task in mice. We find that layer 2/3 neurons encode sensorimotor prediction errors (SmPEs), and critically, we identify muscle spaces as the coordinate framework. To do this, we developed a novel 50-muscle model of the adult mouse forelimb that is capable of studying motor control and learning in a physics-simulator. Using model-derived features, we find that during adaptive learning, functionally distinct neurons are mapped onto specific computational motifs. S1 neurons more prominently encode SmPEs, and the neural latent dynamics change in S1 (but not in motor cortex; M1) during this within-session learning. Together, our results provide a new model of how neural dynamics in S1 enables adaptive learning.
12:30 – 15:00
Posters, Exhibitors and Lunch
15:00 – 17:00
Panel IV - The role of Basal Ganglia in complex walking: Implications for aging and neurodegenerative diseases
Shuqi Liu 1, Julia Choi 2, Caterina Rosano 4, Katrina Nguyen 3
1 University of Pittsburgh, 2 University of Florida, 3 University of Colorado Anschutz Medical Campus, 4 Dorothy Dillon Eweson Lecturer on the Advances in Aging Research
Discussant: Gelsy Torres-Oviedo
Mobility deficits such as reduced locomotor adaptations and dual-task abilities are common with aging and can represent prodromal signs of neurodegenerative diseases including Parkinson’s disease (PD) and Alzheimer’s disease and related dementias (ADRD). In fact, mobility decline often precedes cognitive symptoms in ADRD. Therefore, it is reasonable to hypothesize that shared neural mechanisms underlie the diseases that are thought to be motor, such as PD, or cognitive, such as ADRD. Our panel will discuss one such potential neural mechanism: the basal ganglia.
The panel includes experts in human and animal locomotor control, aging, and neurodegenerative diseases. We will show behavioral, electrophysiology, and neuroimaging evidence on the role of basal ganglia underlying complex walking behaviors, the influence of aging, and implications in patients with PD or Mild Cognitive Impairment (MCI), an early stage of ADRD.
Caterina Rosanowill discuss results from an epidemiological study showing that striatal dopaminergic neurotransmission, measures through neuroimaging, predicts gait speed changes during dual-tasks in older adults, independent of age, sex, and physiological factors such as muscle strength or joint pain. The mechanisms underlying this relation and its therapeutic implications will be discussed.
Shuqi Liu will present data linking two behaviors critical for mobility, dual-task walking and locomotor adaptation, in young and older adults with or without MCI. She will argue that walking tasks that are typically automatic and controlled by subcortical structures like the basal ganglia will require cortical compensations with aging, but the availability of the compensation is impacted by cognitive health.
Julia Choi will present locomotor adaptation data in PD patients with Freezing of Gait (FoG). Behavioral and electrophysiological data from patients who underwent Deep Brain Stimulation (DBS) in the globus pallidus internus (GPi) show that impaired walking adaptation is related to distinct GPi oscillations in PD with FoG. She will discuss how combining bidirectional DBS with locomotor adaptation can provide insights into the brain activity underlying gait dysfunction in PD.
Katrina Nguyen will show locomotor adaptation results from mice with normal vs. pathological dopaminergic circuits. She will revisit classic locomotor paradigms in freely behaving mice, while leveraging new tools for detailed body pose estimation to assess how movements evolve longitudinally in healthy and unilaterally dopamine depleted mice. She will share data for pathological behaviors that emerge and normal behaviors that recover over the course of learning.
In sum, we will show that basal ganglia dysfunction could underlie neurodegenerative diseases predominantly observed in older populations and have both motor and cognitive symptoms, and discuss the potentials of these non-invasive approaches for early detection and interventions in PD and ADRD.
17:00 – 17:30
Members' Meeting
Join us to learn more about the society, the financial position, incoming board members and more!
18:30
Grab a bus and head into Casco Viejo! Space available for the first 100 participants.
08:00 – 10:00
Panel V - Sensorimotor control of the tongue during feeding and voluntary sequences
Nicholas Hatsopoulos 1, John Barrett 2, Ellen Lumpkin 3, Dan O’Connor 4
1University of Chicago, 2 Northwestern University, 3 University of California, Berkeley, 4 Johns Hopkins School of Medicine
Discussant: Callum Ross
The tongue is an important structure for organismal survival because it mediates the critical functions of feeding and, in some species, communication. The neural control of the tongue in human speech has received much attention; in contrast, the neural control of the tongue and associated orofacial structures during feeding is understudied, especially when compared with control of the limbs and eyes. The tongue is particularly interesting because it is a mid-line, soft body hydrostat that possesses no bones or joints and yet its movement and shape can be exquisitely controlled. What is the role of sensory feedback in guiding tongue movements and posture, and what is the role of cortex in this control and coordination with other organs, such as jaws and hands? Nicho Hatsopoulos will begin by presenting evidence that orofacial areas of primary motor (M1of) and somatosensory (S1of) cortices carry detailed information on tongue kinematics during feeding in macaque monkeys. By recording implanted marker kinematics using a novel 3D x-ray video radiography technology together with single unit activity from electrode arrays implanted in M1of and S1of, he will show how neural population activity from these areas can decode kinematic and shape variables across the feeding sequence with accuracies comparable to those of the arm and hand. Ellen Lumpkin will discuss the role of the sensory periphery in feeding by focusing on mechanosensation of the tongue and palate. Using neuroanatomical and calcium imaging approaches, she has found that the complement of trigeminal mechanosensory neurons innervating the tongue is distinct from those innervating palate and skin. In particular, the majority of lingual mechanosensory neurons respond transiently to dynamic stimuli whereas the palate is densely innervated by slowly adapting, pressure-sensitive afferents that innervate epithelial Merkel cells. John Barrett will expand on the functional role of the tongue by considering how it can be used to reach for and retrieve food pellets by coordinating its movements with the jaw and hand in mice. He will characterize the motor syntax of the tongue consisting of an initial reach, deformation of the tongue tip around the pellet, retraction, and finally securing the pellet by coordinated action of the tongue, jaw and hands. He will present on-going work examining neural activity patterns in orofacial and hand primary motor cortices together with 3D kinematic tracking of the tongue and hand to understand how the cortex mediates this coordinated behavior. Finally, Dan O’Connor will present results on the sensorimotor control of voluntary tongue sequences with mice trained to perform “sequence licking” tasks. He will focus on how ascending sensory signals from tongue mechanoreceptors and jaw muscle spindle afferents are integrated with motor signals from regions of primary somatosensory and motor cortices to guide flexible, goal-directed tongue/jaw movements.
10:00 – 10:30
Coffee Break
10:30 – 12:30
Panel VI - Single-neuron dynamics: Unveiling the single-cell type underpinnings of behavior, disease phenotypes, and therapy in motor disorders
Marco Capogrosso 1, George Mentis 2, Kimberly Dougherty 3, Claudia Kathe 4, Thomas Hutson 5, Serena Donadio6
1 University of Pittsburgh, 2 Columbia University, 3 Drexel University, 4 University of Lausanne, 5 Wyss Center for Bio and Neuroengineering, 6 Rehab and Neural Engineering
Discussant: Marco Capogrosso
With the emergence of high-count neurotechnologies and new mathematical tools we’ve been witnessing the development of population neural dynamics as a central tool to study motor control. This framework seems to overcome the limitations on the analyses that can be conducted at a single neuron level to provide insights on the computations executed by large neural networks during motor planning and execution. However, while we enjoy the explosion of this scientific framework, we shouldn’t neglect that single neuronal types have profound impact on behavior, particularly in specialized circuits. Indeed, neurons are not static input-output functions, but rather complex biochemical structures that adapt in response to changes in the environment. In this panel, we will explore how adaptations of single neuronal types in the spinal cord underpin the emergence of motor phenotypes in major motor disorders. We will show how single-cell type changes drive motor recovery and how they can be targeted to develop therapies. To build our case we present a 5 speakers panel divided in two sessions: a basic science session and a new therapies horizon session. In the basic science session Dr. Mentis will demonstrate how the adaptation of a single ion channel in motoneurons is responsible for widespread muscle weakness and atrophy in Spinal Muscular Atrophy (SMA), a progressive motoneuron disease. Importantly, Dr. Mentis will introduce the idea that these changes are driven by homeostatic adaptation rules that re-shape the function of motoneurons in response to SMA-altered circuits. Following this path, Dr. Dougherty will introduce her most recent work in Spinal Cord Injury (SCI). Using a combination of ex-vivo electrophysiology and single-cell type manipulation in mice she will demonstrate that SCI dramatically changes the membrane properties of inhibitory interneurons in the dorsal horn that govern presynaptic gating of sensory afferent and may lead to the emergence of overt hyperreflexia. Finally, Dr. Kathe will show how a group of interneurons in the spinal cord that participate but are not critical to movement in intact mice become necessary components of motor recovery after SCI. The New Therapies Horizon builds on these insights to propose new therapies that target these single-neuron type level changes to improve behavior. Specifically, PhD-candidate Donadio will show how electrical stimulation of the primary afferents can be used to reverse the SMA-driven changes in ion channels of spinal motoneurons and significantly improve strength and fatigue in humans with SMA. Finally, Dr. Hutson will present the proof-of-concept of an optogenetic stimulation therapy that targets hyperexcitable motoneurons after SCI to treat hyperreflexia and spasticity. Discussion with the audience will focus on how single neuronal cell types shape motor behavior and if this can be leveraged to build the new therapies
12:30 – 15:00
Posters, Exhibitors and Lunch
15:30 – onwards
Free time or excursions
Get out and experience Panama! Further information regarding excursion options can be found on the Excursions page.
08:00 – 10:00
Panel VII - Computational mechanisms underlying contextual modulation in motor learning
Tianhe Wang 1, Apoorva Sharma 2, Kahori Kita 3, Daniel Wolpert 4
1 University of California, Berkeley, 2 Yale University, 3 Johns Hopkins University, 4 Columbia University
Discussant: Nobuhiro Hagura
Understanding the role of context is a central issue in learning and memory. Even simple learning processes, such as classical conditioning, rely on forming associations with relevant contextual cues. Over the past 15 years, context has garnered considerable attention in sensorimotor learning research. While traditional computational approaches incorporating meta-learning mechanisms into Kalman filters and state-space models to capture some of the contextual effects, they often struggle to explain the flexibility of motor learning in diverse environments. In contrast, emerging computational methods, including hierarchical Bayesian frameworks and artificial neural networks, have successfully accounted for a broad range of phenomena in motor learning, habit formation, and decision making, substantially advancing our understanding of how context shapes learning. These advances have also fueled new research into the role of context in various motor learning processes, prompting debate on various issues such as whether different sensorimotor adaptation processes share similar contextual modulation, whether contextual effects extend beyond basic adaptation to more complex motor skills, and what neural mechanisms underlie these contextual modulations.
This session will try to address these questions by bringing together researchers who employ a variety of behavioral tasks and computational frameworks. Wolpert will review the role of contextual inference in motor learning and show how contextual inference may underlie the differences in motor learning under distinct training curricula. He will also describe the role of visual and dynamic inputs on the way motor learning tasks are decomposed. Wang will introduce a cerebellar-like network that captures a wide range of contextual effects in implicit adaptation without relying on explicit contextual modulation. He will also present empirical findings that distinguish two distinct implicit components in sensorimotor adaptation, each displaying unique contextual sensitivities. Sharma will demonstrate how internal timing can serve as a contextual cue to separate implicit motor memories in a dual-adaptation paradigm. Kita will examine the retention and spontaneous recovery of newly learned motor skills following extended breaks, exploring how principles of contextual modulation may generalize to de novo learning. Finally, Hagura will lead a panel discussion addressing key questions, including how different processes, such as implicit recalibration and explicit aiming, are modulated by context in potentially distinct ways, and what neural mechanisms support these contextual modulations in motor learning.
10:00 – 10:30
Coffee Break
10:30 – 12:30
Individual III
OS3.2 – Determining the cognitive contributions to reduced movement vigor in people with Parkinson’s disease
Jonathan Wood 1, Amanda Therrien 1, Aaron Wong 1
1 Thomas Jefferson University
Presenting Author: Jonathan Wood
Whether and how we move depends on a tradeoff between the effort required and the payoff (i.e., reward) we receive from moving. Impairment of this tradeoff has been hypothesized to underlie reduced movement vigor (i.e., bradykinesia) in Parkinson’s disease (PD), although the exact nature of this disruption remains unclear. As dopamine has long been linked to reward, its reduced availability in PD could result in a devaluation of perceived reward outcomes. Alternatively, people with PD may perceive moving as excessively effortful. A third possibility is that PD disrupts the mapping between effort and reward. We assessed these alternative hypotheses in 3 separate tasks in people with PD ON and OFF their dopamine medication and compared them to matched controls. Determining the specific source of impairments in the effort/reward tradeoff is critical to understanding movement vigor more broadly and why bradykinesia arises in people with PD.
We first assessed reward perception using a standard behavioral economics task in which participants chose between a certain or risky monetary reward. Reward perception was quantified as the difference in the expected value of certain and risky choices where a person was equally likely to choose either option. Surprisingly, we found no effect of disease (PD ON vs controls p=0.59) or medication status (PD ON vs OFF p=0.36) on reward perception. Contrary to prior theories of dopamine and reward processing, this finding indicates that PD may not significantly impact the perception of reward.
Next, we assessed effort perception using an isometric force-matching task. Here, people were cued to produce a given force magnitude and then attempt to reproduce the same perceived force without cues. While everyone could accurately produce the cued forces, the reproduced forces for people with PD were smaller than for controls (p=0.03), suggesting that people with PD perceived their exertions to be more effortful (and hence did not need to push as strongly to reproduce the perceived force). However, we did not find an effect of medication status (p=0.49), suggesting this perception is not contingent on dopamine availability.
Finally, we assessed the mapping between effort and reward by measuring participants’ willingness to exert a given force for a given reward. Specifically, we quantified the change in force preference as a function of reward magnitude. While controls were willing to exert greater forces compared to people with PD regardless of reward magnitude (p=0.004), the change in force preference across rewards was similar for both groups (p=0.40) and for people with PD ON vs OFF medication (p=0.58). Thus, the effort/reward mapping was not significantly modulated by PD or dopamine. Overall, this work surprisingly hints that reward perception may not contribute to bradykinesia in people with PD. Rather, the perception of effort may play a critical, and previously underappreciated role in movement vigor.
OS3.3 – Walking toward riches: reward pays the cost of effort
Chadwick Healy 1, Alaa Ahmed 2
1 University of Colorado, 2 University of Colorado, Boulder
Presenting Author: Chadwick Healy
Slowing of movements is a symptom of numerous motor and psychiatric disorders, yet our understanding of what determines movement speed is not well characterized. The preferred speed of walking is thought to be determined primarily by energetic cost, but can reward influence walking speed? Recent findings have shown that the speed at which we move reflects the value of what we hope to acquire in eye movements and arm reaches, suggesting a link between the neural processes that control movements and those that assign value. People reach and saccade faster to objects that promise greater reward and when there is greater opportunity cost of time. However, there is currently little understanding of how upcoming reward and a history of reward influence walking speed, a measure often thought to be prescribed by energetic cost alone.
Here, we integrate a self-paced treadmill with a virtual reality system to immerse subjects in a realistic environment that probes the effects of reward, reward history, and effort on walking speed. Sixteen subjects (n = 16; 7 Females; 22.4 ± 4.95 yrs) completed two sessions with different baseline effort conditions. In the high effort session, subjects donned a weight vest with approximately 15% of their body mass. During each session, subjects completed a series of walking trials in virtual reality that involved walking along a path collecting varied values of rewards visualized as apples. We manipulated the value of the rewards (high/10-apples, medium/5-apples, and low/1-apple), as well as the history of reward by changing the average payout of an environment. A rich environment had a higher probability of high reward (50% high, 30% med., 20% low), and a poor environment had a higher probability of low reward (20% high, 30% med., 50% low). Subjects walked at their own pace through the virtual world, where they encountered a series of rewards. Every 40 meters, a cue appeared 30 m ahead, the value of the reward was revealed after walking 10 m further, then subjects walked 20 m further to collect the reward.
Walking speeds increased as the revealed upcoming reward value increased (P < 0.0001). For the same upcoming reward value, speeds were faster in a rich environment than a poor environment (P = 0.0107). Even when the value of the reward was not revealed, subjects walked at faster speeds in a rich environment (P = 0.0119), suggesting there is an opportunity cost of time. In addition, subjects walked slower in the high effort condition versus the low effort (P = 0.0278), confirming that movement speed considers both the reward and effort of a movement rather than either one alone.
Our findings are the first to show that walking speed is sensitive to explicit reward and reward history and is modulated by effort, building on recent findings that both reward and effort can modulate movement speed. These results show significant promise that walking speed can provide a non-invasive marker of cognitive function and motivation.
OS3.4 – Reward influences movement vigor through multiple motor cortical mechanisms
Adam Smoulder 1, Patrick Marino 2, Emily Oby 3, Sam Snyder 2, Steven Chase 1, Aaron Batista 2
1 Carnegie Mellon University, 2 University of Pittsburgh, 3 Queen’s University
Presenting Author: Adam Smoulder
When greater rewards are at stake, humans and animals alike tend to quicken the speed and latency of movements without sacrificing their accuracy – that is, they act with enhanced vigor. How does the brain translate changes in motivation into increased movement vigor? Rhesus monkeys performed a delayed reaching task in which we cued the reward (Small, Medium, or Large) that would be given upon trial success. In population recordings from primary motor cortex (M1) and dorsal premotor cortex (PMd) we identified multiple neural correlates of motor vigor affected by reward.
First, we observed correlated effects of reward magnitude and upcoming movement vigor on movement preparatory neural activity. We identified a “vigor axis” in neural population space whereby the response of the motor cortex population correlated with movement vigor on a trial-by-trial basis. We found that greater rewards positively modulated activity along the vigor axis. We also observed that greater rewards made reach-direction conditions more separable in neural activity, and greater separability correlated with greater vigor.
Second, we found that reward facilitated the transition from preparation to movement. We calculated a neural changepoint time for each trial and saw that it decreased with greater reward. We also found that the neural speed (time derivative of firing rates) preceding movement onset was faster for greater rewards. Both of these metrics were correlated with the animals’ movement vigor.
Third, we saw that reward altered neural trajectories during movement execution. Greater reward appeared to “stretch” trajectories and quicken the traversal of neural activity along them. These effects imply gain-like effects of reward on motor cortical activity, hinting at potential underlying mechanisms of the effects we observed (e.g., neuromodulatory drive).
We considered that these motor cortical correlates between reward and vigor might be driven by a single source. In this case, we would expect neural correlates of vigor to exhibit strong correlation structure themselves. Instead, we observe limited correlations between the metrics, implying multiple aspects of motor cortical activity both affect movement vigor and are modulated by reward.
Lastly, we note that when we controlled for vigor across reward conditions, we saw little-to-no impact on the ability to decode the reward cue from the neural activity. This indicates that reward effects on motor cortical activity far exceed those we would expect from reward-mediated differences in movement vigor, and that motor cortex does not simply encode reward information that is directly relevant to behavior.
We conclude that reward influences multiple aspects of motor cortical activity that relate to movement vigor, and that reward effects on motor cortex exceed those that can be explained by differences in behavior.
OS3.6 – Understanding surprise: Dual predictive systems in whisker sensorimotor control
Ritu Roy Chowdhury 1, Kalpana Gupta 1, Yuyao Sun 1, Franziska Gekeler 1, Shubodeep Chakrabarti 1, Cornelius Schwarz 1
1 Eberhard Karls University of Tübingen
Presenting Author: Ritu Roy Chowdhury
The predictive coding framework suggests that the brain continuously generates and updates predictions to minimize sensory prediction errors, but we still don’t fully understand how these predictive mechanisms relate to movement. We sought to systematically separate two systems that attenuate movement-generated sensory flow—sensory gating (SG) and state estimation (SE). We hypothesized that SG applies a broad temporal filter to movement-related sensory consequences, while SE selectively attenuates expected sensory inputs at specific times, thereby amplifying relevant inputs.
We recorded S1-spiking effects in mice performing a whisker-reach task, adapting Curtis Bell’s 1981 ‘open-loop’ paradigm from fish to mammals. First, we investigated SG’s temporal profile while maintaining a fixed probability of sensory consequences. Next, we increased the probability of sensory inputs at specific time points to characterize SE-driven attenuation patterns. We show for the first time that SG peaks around movement onset, while SE disrupts this profile by producing a new peak of sensory attenuation around expected time points. Additionally, we found that SE helps to suppress whisker movements evoked by surprising sensory inputs.
Optogenetic manipulations suggest that the cerebellum plays a key role in SE-induced sensory attenuation but not in the one induced by SG. Together, these findings advance our understanding of how the brain uses multiple predictive mechanisms to optimize sensorimotor control and behavior.
12:30 – 15:00
Posters and Lunch
15:00 – 17:00
Panel VIII - Inter-area communication for motor control
Emily Oby 1, Matthew Perich 2, Sam Snyder 3, Stefan Lemke 4, Maureen Hagan 5
1 Queen’s University, 2 Université de Montréal, 3 University of Pittsburgh, 4 University of North Carolina, 5 Monash University
Discussant: Emily Oby
Motor control is remarkable in its flexibility- allowing us to perform a wide range of complex movements, adapt to new tasks, and choose appropriate action for a given context or environment. The brain achieves this flexibility, at least in part, through the dynamic interactions between distinct brain areas in the sensorimotor loop. From the sensory cortices processing incoming information, to the motor areas executing precise movements, and the higher-order brain areas responsible for planning and decision-making, each area interacts and communicates with other areas in order to orchestrate the movement. How is communication instantiated and modulated across brain areas to allow for flexible motor control? In this session, we will explore recent work that examines how the sensorimotor brain areas coordinate and communicate their activity, enabling flexible and precise motor control.
Neural communication must be dynamic and flexible in order to guide behavior. Matt Perich will explore methods to estimate principles of inter-areal communication and demonstrate that transient interactions between sensory and motor areas can enable flexible behavioral output.
Behavioral tasks like eye-hand movements engage brain areas across networks to coordinate behavior and can allow us to study neural mechanisms of communication across brain areas. Maureen Hagan will show how the temporal patterns of neural activity across areas is modulated by behavioral demands and may be a signature of inter-area neural communication.
Precise, genetically-defined manipulators of cortical, subcortical, or cerebellar areas can each disrupt skilled motor control in rodents. However, the manner by which these distributed areas may interact to control skilled movements remains unclear. Stefan Lemke will present evidence that the strength of cross-area coordination captured from large-scale neurophysiology during motor control covaries with the ability to perform skilled movements in genetic mouse models with typically and atypically developed nervous systems.
Finally, Sam Snyder will share a causal test of the flexibility of interactions between primary motor (M1) and premotor cortex (PMd). Using a brain-computer interface task designed to challenge monkeys to break observed correlations between M1 and PMd, he will show that inter-area interactions are more flexible than within area interactions.
Together this panel will highlight the importance of understanding how distinct populations of neurons interact and communicate to plan, execute, and learn new movements. The discussion will focus on our understanding of how the brain flexibly controls movement and if this can shed light on how disruptions to the sensorimotor neural networks might contribute to motor impairments.
17:00 – 18:00
Distinguished Career Award Presentation - Richard Ivry
The cognitive neuroscience revolution provided a new theoretical framework for using the tools of neuroscience to study the mind. Central to this paradigm shift was the idea that the focus of our analysis should be on the component operations underlying task domains rather than on the tasks themselves. I will provide a retrospective on our efforts to apply this strategy to motor control, and in particular to develop a functional account of the role of the cerebellum in coordinated movement, sensorimotor learning, and cognition. Using simple model tasks, the behavioral analysis of healthy individuals and patients with cerebellar disorders has provided insight into unique computational characteristics of this subcortical structure. Prominent among these is the idea that the cerebellum is critical for tasks that require the precise representation of temporal information. This hypothesis places an important constraint on the predictive capacity of the cerebellum, providing an account across a range of task domains of conditions that are cerebellar dependent and, as important, conditions that are cerebellar independent. These dissociations are essential for building a psychological level account of how the cerebellum supports coordinated movement and thought.
18:00 – 19:00
Closing Drinks Reception
Following the Distinguished Career Award presentation and talk, join us for a drink to celebrate NCM 2025!